Various means of storage are used to make the grid more flexible: the electricity is stored during periods of excess production and output to meet consumption needs. These means include:
- hydraulic storage by STEP (pumped energy transfer station);
- mechanical storage by inertia flywheel;
- electrochemical storage;
- storage by heat;
- chemical storage;
- superconducting inductance storage.
Hydraulic storage is the most widespread solution. However, few sites are available in France to accommodate new capacity. Inertia flywheels are used to meet high power demands for short periods. These two solutions are mature and are no longer the subject of major research work. Magnetic inductance storage which consists in storing energy in magnetic form thanks to the use of superconducting coils is still at the pre-industrial demonstration stage and little research is being carried out. The most closely examined technologies today for short to medium term applications are electrochemical, chemical (hydrogen) and thermal storage solutions.
Electrochemical storage
Stationary storage of electricity in batteries is a solution already extensively deployed in a certain number of countries. The vast majority of the installed capacity is based on lithium-ion battery technology. This is the technology of choice owing to its rapidly falling cost owing to growing demand from the electric vehicles sector. France has an ambitious roadmap with the goal of installing 1GW/1.5GWh of storage capacity by 2023. This goal is reinvigorating the storage sector with several French players at the forefront: battery manufacturers, integrators, recyclers, etc.
For several years now, CEA has been engaged in a research programme to make stationary storage by batteries deployable to the national and also the export market. This programme comprises several aspects on different time scales: develop integration of Li-ion cells in a pack equipped with a smart management system, for the short-term; search for an alternative technology to conventional Li-ion offering better power and cyclability performance, for the medium-term; prepare disruptive technologies to allow a significant increase in energy densities, for the long-term.
Smart pack
Using a battery as a stationary means of storage might be considered an extra cost given that other storage solutions exit. Considerable work is thus being done to bring down the cost of the energy carried in the pack (called LCOS for Levelized Cost of Storage). This cost includes not only the initial investment in manufacturing the pack components (CAPEX), but also the cost of their maintenance (OPEX) and takes account of the number of kWh output over the lifetime of the system. Several avenues are being explored to bring down the LCOS:
- bring down CAPEX more specifically by working on the equipment associated with the cell and making up the pack (converter, cooling system, etc.) and investigate the principle of a second life for the batteries;
- minimise OPEX by developing diagnostic tools able to conduct preventive maintenance;
- increase the kWh output by lengthening the lifetime (optimisation of materials and management of the battery during utilisation).
Achieving these three goals requires complete understanding of the performance losses by the various components making up the pack, in order to define strategies to remedy them. Considerable work has thus been undertaken to study the deterioration of the components, combining modelling, testing and advanced characterisation.
Numerous off-the-shelf cells have been tested in recent years on the test benches at INES, to measure their performance loss in various conditions: calendar ageing (at rest), in operation, with various charge/discharge levels, at various temperatures or with different charge regimes (fig. 1). The cells are “autopsied” after the tests to determine the mechanisms responsible for their deterioration. We for example identified the formation of lithium metal during calendar ageing of charged cells at a temperature of 45°C, following the appearance of bubbles resulting from the decomposition of a component of the electrolyte (fig. 2). Simulation calculations confirmed the hypothesis of the mechanism involved postulated as a result of the characterisations. The component responsible for this degradation was identified and the manufacturer is seeking to replace it by a more stable additive.

Fig. 1: Illustration of endurance performance tests showing the evolution of the state of health (SOH) of several families of Li-ion batteries during ageing with cycling at 1C/1C, 45°C, 100% discharge depth.
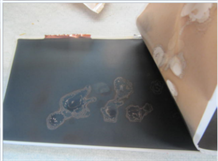
Fig. 2: Observation of deposits of lithium metal located around concentric zones
Management System (or BMS). The BMS is a complete system comprising measurement and balancing functions, battery status and safety algorithm and also active control. Research is focusing on management algorithms and on the power electronics. The BMS can also contribute to improving the safety of the packs, as the batteries entail a risk of thermal runaway. With (real or virtual) sensors capable of detecting temperature rises or early warning signs of runaway (internal short-circuit by dendrite growth) at an early stage, it is then possible to trigger protection actuators, for example by bypassing the defective cell or by activating a cooling system.

Fig. 3: Assembling a battery pack © Patrick Avavian/CEA
The alternatives to conventional Li-ion
One of the technologies currently being studied concerns the use of sodium ions and the NVPF/Hard Carbon electrochemical pairing. This technology is an interesting alternative in terms of power and cyclability, making it potentially interesting for stationary applications. CEA is working on the development of this technology in close collaboration with the academic partners of the RS2E network. Industrial format storage cells have already been produced (fig. 4). Although initial results would appear to be highly promising, work must be continued to qualify this new technology and optimise manufacturing costs. For the longer term, other technologies are envisaged, such as the lithium – metal family which offers far greater energy densities but which runs the risk of short-circuits owing to the formation of metal dendrite during prolonged cycling, especially if the charge regime is high. New organic redox flow type technologies are also being studied for stationary applications. France has a player well-placed in this segment, which recently developed a first 10 kW demonstrator.

Fig. 4: Sodium-ion storage cell © Vincent Guilly/CEA
Mobility-stationary convergence
Electrical recharging stations covered with solar panels have been installed in Corsica (fig. 5). The advantage of these stations is to offer 100% solar recharging: vehicle consumption is covered in full by solar production. To achieve this goal without having to resort to high-capacity batteries in order to independently manage the time lag between production and consumption, the choice was made to opt for low-capacity batteries and connect the stations to the existing electricity grid. A smart management system runs the network of stations, making the decision between injection into the grid and storage in the stationary batteries, so that in the end, the 100% goal is achievable with a sufficient number of stations.
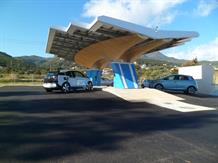
Fig. 5: Parasol station in Bastia, collaboration with Driveco
Chemical storage with hydrogen
When used as an energy carrier, hydrogen brings flexibility to the electricity grid. In the event of surplus electricity, it is used to produce hydrogen by electrolysis of water. The hydrogen generated is then stored in gaseous form in pressurised tanks, or in solid form in hydrides (technology currently being developed). It can then be used for industrial applications, consumed locally to power hydrogen vehicles, injected into the natural gas network, combined with CO2 to generate synthesised methane, or transformed back into electricity by a fuel cell. This latter case is envisaged for areas said to be non-interconnected, in other words which cannot be connected to the grid.
CEA is a partner in several projects to test these different uses of hydrogen. One example is the JUPITER 1000 project (fig.6), the aim of which is to demonstrate the economic viability of the complete “Power To Gas” concept at MWe scale: production of hydrogen in its electrolysers supplied with electricity of 100% renewable origin, transformation into synthesized methane and combination with the CO2 in the smoke from the nearby plant, with injection of this methane into the natural gas network.
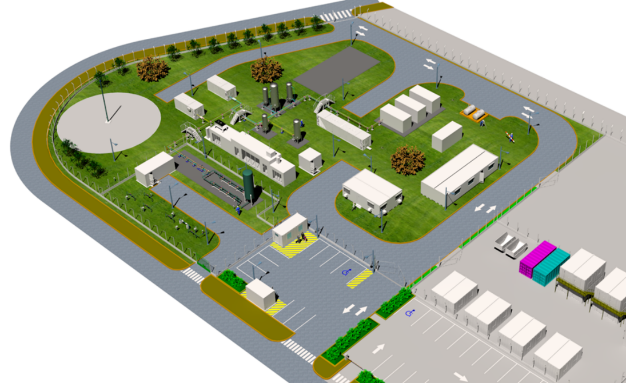
Fig. 6: mock-up of the JUPITER 1000 site
Storage through heat The large-scale storage of electrical energy in the form of heat has been envisaged for many years, either as a secondary contributor, or as the main carrier. The advantage of this solution is the simplicity of sensible heat, in other words a hot liquid or solid. The main obstacle to its development is the low conversion efficiency of heat to electricity (about 30% with considerable dependence on the temperature level). Different approaches are then possible: improve the overall efficiency of the process (electrical to electrical) by working with a “heat pump”, or attempt to bring down equipment costs to make such low efficiency acceptable.
With the aim of limiting costs, we propose rock bed sensible heat storage. This is the option chosen for a technological concept developed under a research project in partnership between the Babcock Watson company and CEA Liten using a thermal oil as heat transfer fluid at 350°C, a 10Mh storage tank (fig. 7), exchangers to vaporise water under pressure and a steam turbine. The efficiency achieved is then about 25%.
Another concept, known as thermal pumping, on the contrary seeks to achieve the highest possible efficiency by using the principle of a very high temperature heat pump (800°C) combined with a gas turbine. In this case, it is possible to achieve efficiency of 65%. This is a reversible closed cycle with higher power turbomachines (100MW) and the working fluid is a neutral gas at several bars of pressure. Heat storage is via two chambers storing the hot point and the cold point of the cycle respectively. It is obviously the highest temperature, 800°C, which determines the choice of the solid material filling the chambers: basalt, which experienced far higher temperatures during its formation process, is ideal.

Fig. 7: Heat storage tank and materials envisaged
Dimensioning of a storage solution
As we can see, various solutions can be used to bring flexibility to the electricity grid. The choice of the technologies and their dimensioning (power, capacity) is the result of multi-criteria optimisation: technical, economic and user satisfaction. CEA has developed tools allowing modelling and simulation of the operation of micro-grids integrating renewable production sources, batteries, a hydrogen chain and the users. The storage systems can be dimensioned in a variety of ways to assess the technical/economic benefits for a system, a function or a given consumption.
For example, we dealt with the case of a port facility in Corsica, for which the advantages of storage were evaluated to maximise self-consumption of photovoltaic energy and minimise the use of the electricity grid at peak hours. The economic viability expressed as updated energy costs (Capex cost + Opex divided by the quantity of energy produced over the lifetime of the equipment, LCE) for solutions using batteries alone or battery/hydrogen hybrids was assessed for various PV fields and a maximum withdrawal from the main distribution grid of 10kW. The technical/economic advantages of hybridisation become apparent when the grid withdrawal constraint is high (fig. 8).
Fig. 8: Results of technical/economic analyses with different storage solutions (lead battery, lithium battery, hybridisation with a hydrogen chain)